Live fast, die young
Wolf Rayet – the very name speaks of something strange and violent. And indeed Wolf Rayet stars are passing strange and meet with a violent end.
Early Discovery
In the middle of the 19th century two astronomers at Paris Observatory – Charles Wolf and Georges Rayet – were working on stellar spectra, when they came across a few stars with a very unusual spectral signature. They found that the spectra had distinct emission bands, which were significantly broadened.
![]() |
![]() |
Most stars have absorption lines in their spectra, where atoms in their outer atmosphere absorb some of the light emitted from the surface of the star. And generally, the absorption lines are fairly narrow and weak. But in the case of these Wolf Rayet stars, the light was being emitted in the outer atmosphere, and the emission lines were much stronger and wider than normal.
There are a number of reasons why spectral lines can be broadened but in the case of Wolf Rayet stars, the answer is that luminous material is being driven off the star at very high speeds in all directions. The strength of the lines indicates that an enormous quantity of material was involved.
In a further peculiarity, there was a hint of an unknown element in the spectrum. The following year (1868) Rayet and other observers clearly saw the spectral lines during a solar eclipse. Jules Janssen and Norman Lockyer are generally credited with the discovery of the new element – to which Lockyer gave the name Helium.
As an aside, we should consider the technology available to Wolf and Rayet in 1867. Astrophotography was in its infancy and photo-spectroscopy was even newer. The first spectrographs of stars other than our Sun were recorded in 1863 by Huggins and Miller – however, the image only showed the continuum spectrum. It was not until 1872 (five years after the WR discovery) that John Draper was able to photograph a spectrum with absorption lines.
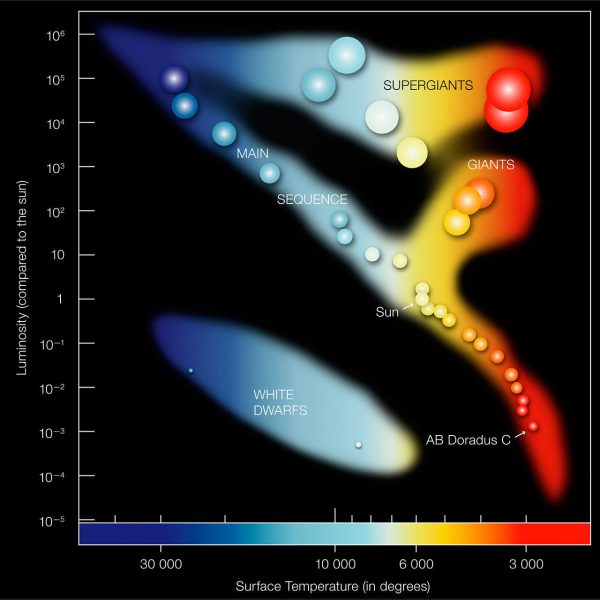
So what kind of star is it that Wolf and Rayet found? What type of star blows of so much matter with so much energy? And why are there so few of them?
The answer is found way up at the top of the Hertzsprung-Russell diagram, where stars are both extremely massive and extremely hot. These O-type stars are thirty or more times the mass of our Sun and burn at enormous temperatures. Towards the end of their lives, massive blue stars go through an evolutionary process that ends when they explode as supernovae. Part of this process (for at least some of these stars) involves a Wolf Rayet phase.
In this phase, much of the hydrogen has been used up and the star is burning helium, carbon, nitrogen and oxygen to form heavier elements. Convection currents bring these elements to the surface of the star where they continue to undergo fusion.
This process leads to the classification of WR stars according to the most prominent spectral lines: WC (carbon, oxygen and helium), WN (nitrogen and helium) and WO (oxygen). There are further sub-sub-classifications of 0 – 9, depending on temperature. So for example a hot helium/nitrogen WR star would be classified WN1.
The more massive a star is, the hotter it burns. A Wolf Rayet star burns extremely hot – not less than 20,000 Kelvin at the surface – developing a prodigious radiation pressure which is enough to blast its outer layers into space as a super stellar wind. This wind carries away mass at the rate of 10-5 solar mass every year. Think about that: in 100,000 years, the entire Sun has been blown away!
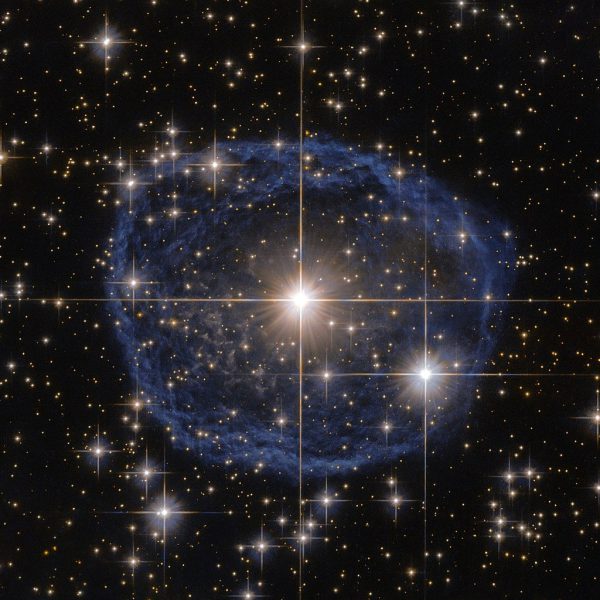
Wolf Rayet stellar winds have been measured at speeds between 800 and 3,000 km/sec. Think about that: Perth to Sydney in a second!
As the winds blast away from the stars, they interact with the interstellar medium, in some cases forming beautiful spherical nebulae, such as WR 31a in Carina. This bubble is about 20,000 years old and is expanding at 60 km/sec – about 220,000 km/h.
More commonly, WR stars are found in binary pairs and the interaction of the stellar winds creates enormous quantities of dust. This obscuring dust, plus the fact that most of the radiation is in the ultra-violet, means that WR stars are generally not good targets for optical telescopes. One exception is γ2 Velorum, the nearest Wolf Rayet to Earth and the only one visible with the naked eye.
We said earlier that WR stars are typically greater than 30 solar masses. In fact many are somewhat less than that, having already blown away an enormous quantity of matter. But some are much, much more. We think of stars like Betelgeuse as being massive, but they have less than ten solar masses. By contrast, the largest WR stars have hundreds of solar masses.
The greatest collection of massive stars is the R136 cluster in the Tarantula nebula. This has some of the most massive stars known – and all are Wolf Rayets.
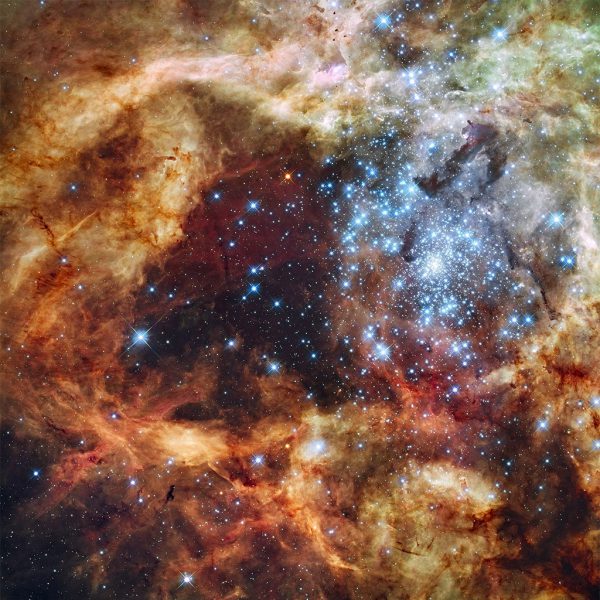
R136a1 is the most massive star known, with an initial mass estimated at 315 solar masses. This enormous star loses mass equivalent to an Earth every 22 days, is eight million times more luminous than our Sun and radiates as much energy in five seconds as the Sun does in a year. It is the most luminous star ever discovered.
How are they born?
WR stars are very rare – less than 200 are known in our galaxy. However, current thinking is that all very massive stars will go through a Wolf Rayet stage at the end of their lives. Because these stars burn so hot, their life spans are extremely brief – and the WR stage is even briefer – so only a few are passing through the WR stage at any given time.
Super-massive stars are very unstable and cannot form directly from a gas/dust cloud. It is thought that they are in fact the product of a merger of two smaller (but still enormous) stars.
How do they die?
WR stars go out with a bang! Depending on the mass and metallicity, there are a number of potential death processes, all of them ending in a supernova or even a hypernova.
For lower mass WR stars, the end comes when fusion at the core produces iron. This element cannot fuse and give off energy, and so the radiation pressure from the core is shut off abruptly, the core collapses and a shockwave tears the star apart. The remnant may be a neutron star or black hole.
For stars between about 130 and 250 solar masses, a totally different mechanism occurs – pair-instability. In very hot massive stars the radiation pressure of γ rays supports the outer layers against gravity. As temperatures increase, the γ rays interact with atomic nuclei, electrons or each other to form electron-positron pairs. Electrons and positrons, in turn, annihilate each other, releasing energy. The production and annihilation of pairs are maintained in a precarious balance until pressures and temperatures increase even further. At this point, pairs are created more rapidly than they can annihilate, reducing the photon pressure available to support the mass of the star. The outer layers fall in, raising pressure even further and a runaway thermonuclear explosion ensues, totally destroying the star. There is no neutron star or black hole remnant – the star’s material is totally dispersed into space.
The heaviest stars die through a process known as photodisintegration, in which heavy atomic nuclei in the star’s core absorb γ rays. The nuclei become excited and break up into simpler elements. This process is endothermic – it absorbs energy – and abruptly reduces the radiation pressure holding the star up against gravity. Again, the star collapses, leaving a black hole remnant.
Like Jimi Hendrix and Janis Joplin, Wolf Rayets are massive stars. They burn bright, live fast – and die young. WR stars are among the strangest and most violent objects known in our universe.